|
Indian Pediatr 2010;47: 127-128 |
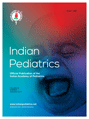 |
Phototherapy: The Challenge to Accurately
Measure Irradiance |
Hendrik J Vreman
Senior Research Scientist, Division of Neonatal and
Developmental Medicine, Department of Pediatrics, Stanford University
School of Medicine, 300 Pasteur Drive, Rm S214, Stanford, California
94305, USA.
E-mail: [email protected]
|
I n a seminal report, about fifty
years ago, Cremer, et al.(1) defined the basic principles of
phototherapy as the spectral distribution for the photo-destruction of
bilirubin (action spectrum ~ 400-520 nm), the effect of light intensity on
the rate of bilirubin photo-degradation in vitro (t˝=23 minutes,
for sunlight) and the in vivo rate of bilirubin decline (–1.0 to
3.5 mg/dL/h or –17 to 60 µmol/L/h) under sunlight. Realizing that the use
of sunlight phototherapy entailed various strategic and clinical
disadvantages, they proceeded to investigate the more easily controlled
light from artificial light sources. Thereupon, the first artificial light
device, with strategically placed reflectors, was thoughtfully developed.
Using eight closely spaced blue (B) fluorescent tubes, they studied the
first overhead treatment of newborns in a cradle. This device demonstrated
a very significant and satisfactory fall in bilirubin (0.08 to 0.50 mg/dL/h
or 1.4 to 8.5 µmol/L/h). Although it took some time for the world to
realize the significance of this novel therapy, it is now the global
standard of care(2-4).
It is now well established that an effective
phototherapy device needs to deliver light with (a) a light
emission spectrum within the bilirubin absorption spectrum (400-520nm); (b)
a peak emission of 450±20nm; (c) an irradiance foot print which
exposes at least one horizontal body surface plane or optimally the entire
circumferential (360ş) body surface area; (d) an irradiance level
ł30
mW/cm2/nm,
as measured with an appropriate irradiance meter; and, (e)
optimized duration of exposure (3-5). Many phototherapy devices with light
sources such as blue and special blue fluorescent tubes, halogen, and
halogen/fiberoptic, and most recently, light emitting diode-based (LED)
lamps have been developed and marketed. However, for use in communities
with constrained resources, these devices are often not affordable to
purchase or to maintain. Hence, a cottage industry of phototherapy devices
has evolved using locally available or adapted light sources such as
daylight- and blue-painted fluorescent tubes and incandescent lamps. Most
of these devices have never been adequately tested for performance or
irradiance delivery. Their safety, efficacy and performance remain a
public health concern.
An earlier in vivo study(5) and our recent bench
data(6) illustrate the dose-response relationships of phototherapy as a
direct relationship between the
irradiance used and the rate at which the serum/plasma bilirubin declines
under blue light phototherapy. Each blue light source typically
emits a specific spectral range of photons with a characteristic peak
emission. Similarly, each irradiance meter typically displays its own,
specific and characteristic spectral sensitivity distribution and peak.
Consequently, light source and irradiance meter need to be matched
carefully by the manufacturer. Thus, meters for specific light source
irradiance measurements are not interchangeable(7). In fact, it has
not yet been demonstrated that even the matched sets of light source and
meter accurately measure irradiance. This situation has given rise to much
confusion regarding levels of irradiance, not only at the bedside, but
also with many research studies, particularly those that compare the
efficacy of different lamps for phototherapy. In many published studies,
if an irradiance value is reported, the light meter is often not described
or identified.
Two major issues impact the use of any light meter; its
ability to measure a phototherapy device’s decay of irradiance over time
and its ability to quantitate the delivered irradiance over the entire
therapeutic spectral range. Though these issues are of technical concern
in a clinical situation, there is an urgent need to standardize their
application in research studies. It is important to be able to obtain
accurate absolute measures of irradiance, especially when irradiance
levels are used as a criterion for comparison of lamps with differing
spectral emission. The best method for this situation appears to be the
use of a calibrated flat response spectrometer for measuring the energy
distribution (µW/cm 2) across an
accurately determined in vivo action spectrum of bilirubin
photo-degradation (~400-520nm). From the integration of spectral range and
energy level, the spectral irradiance can then be calculated in terms of
µW/cm2/nm. However, this type of spectrometer system needs to be assembled
by experts for this specific purpose and is not available for bedside use.
Kumar, et al.(8) also faced an
irradiance-related dilemma when they set out to compare the efficacy of
phototherapy with compact fluorescent tube-based device vs a light
emitting diode device. Instead of normalizing the two devices on the basis
of irradiance, the most common method, they elected to normalize devices
on the basis of equal distance of light source to patient (25cm). In doing
so, they found that both devices were equally effective, even though the
irradiance footprints and irradiance levels, measured with the Fluoro-Lite
451 meter (Minolta/Air-Shields, USA) (a functional equivalent to the
Ohmeda Medical Bili Blanket meter) at one of the three study centers,
varied dramatically. The title of their study implies that the study goal
was to compare two different light sources. In retrospect, it would have
been more appropriate, to normalize both devices with respect to
irradiance and its footprint. However, dedicated light meters for the
light sources used for the study were unlikely to have been available.
Instead, the authors have done the best they could under the
circumstances, by measuring the irradiance of both light sources with a
well-identified irradiance meter, so that their experiments can be
reproduced, should that be desirable.
Clearly, towards improving the application of effective
phototherapy, there is an urgent need to develop an affordable,
user-friendly, handheld, universal irradiance meter which accurately
measures irradiance delivered by all types of phototherapy light sources.
Funding: None.
Competing interests: None stated.
References
1. Cremer RJ, Perryman PW, Richards DH. Influence of
light on the hyperbilirubinemia of infants. Lancet 1958; 1: 1094-1097.
2. Subcommittee on Hyperbilirubinemia. Manage-ment of
Hyperbilirubinemia in the Newborn Infant 35 or More Weeks of Gestation.
Pediatrics 2004; 114: 297-316.
3. Philip AGS, Lucey JF. Historical perspectives:
Phototherapy. NeoReviews 2003; 4: 27.
4. Maisels MJ, McDonagh AF. Phototherapy for neonatal
jaundice. N Engl J Med 2008; 358: 920-928.
5. Tan KL. The pattern of bilirubin response to
phototherapy for neonatal hyperbilirubinemia. Pediatr Res 1982; 16:
670-674.
6. Vreman HJ, Wong RJ, Murdock JR, Stevenson DK.
Standardized bench method for evaluating the efficacy of phototherapy
devices. Acta Paediatr 2008; 97: 308-316.
7. Vreman HJ, Wong RJ, Stevenson DK. Phototherapy:
current methods and future directions. Semin Perinatol 2004; 28: 326-333.
8. Kumar P, Murki S, Malik GK, Chawla D, Deorari AK,
Karthi N, et al. Light-emitting diodes versus compact fluorescent
tubes for phototherapy in neonatal jaundice: a multi-center randomized
controlled trial. Indian Pediatr 2010; 47: 131-137. |
|
 |
|